- Published 24 Jan 2023
- Last Modified 12 Mar 2024
- 10 min
LED It Grow: Using LEDs to Boost Plant Growth

Published January 2022
By 2050, the world’s population is expected to hit 9.7 billion. It’s down to agriculture to feed all these people while making very careful use of the resources of soil, water and energy.
One solution for this is LED horticulture. This technique uses covered cultivation areas without the need for sunlight. It is based on the realisation that plant growth can be optimised with the targeted use of coloured light from light-emitting diodes.
LED lights are energy-saving and indirectly minimise the use of pesticides and fertilisers as well as the consumption of water and land by crops. Transport routes can be shortened by erecting indoor greenhouses near urban centres, in turn cutting CO2 pollution. By way of example, 98% of the lettuce grown in the US comes from California and Arizona. Trucks and freight trains have to travel up to 4,200 km to get the green leaves to consumers in New York. As transportation costs have a big impact on the selling price, the urban farming LED concept could even make vegetables cheaper.
Additionally, supply trends such as urban farming or vertical farming are only possible with the crucial lighting provided by LEDs.
The Cost Factor
The most important light source for growing indoor plants used to be high-pressure sodium-vapour lamps. However, LED grow lights are more energy-efficient, generate less heat, and have a longer service life (Table 1 and Table 2).
Table 1: High-Pressure Sodium Vapour:
Light Source | Input Power (W) | PPF (µmol s-1) | Photon Efficiency (µmol J-1) |
---|---|---|---|
400W (Magnetic) | 443 | 416 | 0.94 |
1000W (Magnetic) | 1067 | 1090 | 1.02 |
1000W (Electric) | 1024 | 1333 | 1.30 |
Table 2: LED (@350mA):
Light Source | Input Power (W) | PPF (µmol s-1) | Photon Efficiency (µmol J-1) |
---|---|---|---|
WL-SMDC Deep Blue | 1.12 | 2.31 | 2.06 |
WL-SMDC Hyper Red | 0.84 | 1.81 | 2.15 |
WL-SMTC Moonlight | 1.12 | 1.58 | 1.41 |
WL-SMTC Daylight | 1.12 | 1.69 | 1.51 |
Tables 1 and 2: Photon efficiency of LED versus HID light sources (Source: Würth Elektronik).
The investment costs of high-pressure sodium-vapour lamps are generally two to four times lower than those of LED systems. However, the return on investment of LED-based lighting can be achieved after just one to two years, as their energy consumption is so much lower. This is called wall-plug efficiency; the ratio between optical power and electrical power, or what percentage of power is converted into light.
The most efficient high-pressure sodium system available can achieve a wall-plug efficiency of more than 50%. The most efficient LED system with a combination of Hyper-Red 616-nanometre LEDs and Deep-Blue 450-nanometre LEDs (these two wavelengths are perfect for horticulture applications) can reach values above 80%.
At the right temperatures (below the maximum operating temperature), LEDs work for up to 60,000 hours, which equates to 9.1, 13.7 and 20.5 years if they are operated for 18, 12 and 8 hours per day respectively. At higher temperatures or when running on higher currents, this figure drops significantly. LEDs can be operated at up to around 70% of their light output.
High-pressure sodium-vapour lamps (1000W) equipped on both sides have a service life of 10,000 to 24,000 hours, i.e. 3.7, 5.5 and 8.2 years with an average use of 18, 12 and 8 hours per day. However, because operators want to comply with the required lumen values, you can expect to have to replace the bulb within the first five years.
Metal-halide lamps have a lifespan of between 6,000 and 20,000 hours, while fluorescent bulbs (T-5 and T-8) have a lifespan of 20,000 to 36,000 hours. Again, due to compliance with certain lumen values, lamps are expected to be replaced before this maximum is achieved.
Horticulture LEDs Colour
Different properties of light work together to control the growth and development of plants. The primary factors are the right spectrum of the light source, the efficiency of the light source, and the light intensity. From an economic point of view, the service life, as well as investment and operating costs of the lighting, play an important role.
White LEDs are still more efficient than high-pressure sodium systems. Although with an outlet efficiency of 66%, this option is well below a direct-emitting red/blue combination.
As plants are immobile, they can only react to irradiating wavelengths and light intensities. This may cause reactions to growth in the shade, during the daytime/night-time or to different climates. Artificial light of varying wavelengths can therefore be used to control or manipulate the growth and developmental stages of plants. The resulting responses include the speed of photosynthesis (photosynthesis rate), the influence on the plant anatomy (photomorphogenesis), and determining the direction of growth (phototropism).
Plant-powered photosynthesis is a process in which light is converted into chemically bound energy. It produces high-energy biomolecules from low-energy substances with the help of light energy. In this process, light-absorbing dyes (pigments) such as chlorophyll help to convert light into chemical energy. This is then mainly used to build carbohydrates from carbon dioxide and water.
Image 1
Photosynthetically active radiation in the PAR range.
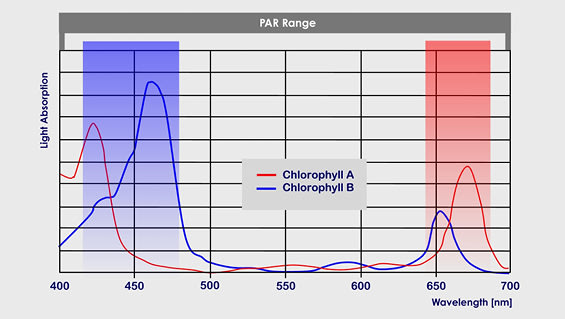
The main pigments are Chlorophyll A and Chlorophyll B. Chlorophyll A is the primary light pigment, which accounts for about 75% of photosynthetic activity and has absorption peaks at 435nm and 675nm. Chlorophyll B extends the wavelength range that can be used for photosynthesis with absorption peaks at 460nm and 640nm. This red/blue range is called Photosynthetically Active Radiation (PAR) and ranges from about 400nm to 700nm (Figure 1). Experiments show that a red/blue spectrum leads to a significantly higher proportion of biomass than the spectrum from white grow lights.
Green light (500 - 600nm) has long been considered unimportant for plant development, but studies have shown that plants in the shade of other plants have a particularly healthy “shade avoidance response” to this wavelength. Light in this wavelength range is usually generated with white LEDs, which also provide blue wavelengths.
In indoor farming, the usual LED combinations are red and blue or red and white. White LEDs “naturally” emit a high proportion of blue, however, they also provide light in the red wavelength range which would usually already be covered by red LEDs in the lighting system. Purely white lighting would require considerably more energy for a comparable number of red photons than directly red-emitting LEDs.
Image 2
Cost pattern when using red and white LEDs.
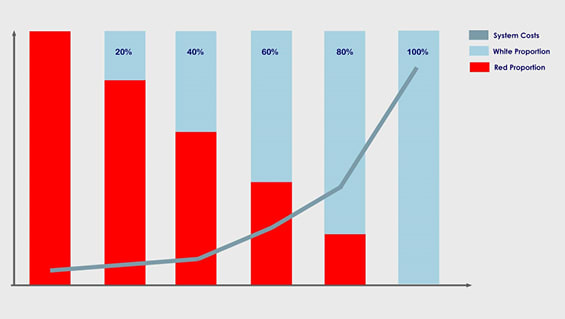
With its Osconiq S 5050, Osram has achieved a higher system yield in the red and white light spectrum by reducing the proportion of phosphor-converted red photons. A special fluorescent material means the metaphorical chop for the red spectral range. It considerably reduces the red proportion of the LED’s white light, and a red/white LED combination would pay off in terms of energy.
Full Spectrum LEDs: Getting the Right Mix
Lights in horticulture must provide a certain amount of photons to ensure efficient plant growth. The required light intensity for different types of plant species varies and must be based on the following values:
- From the power of a light source in PPF (Photosynthetic Photon Flux) – the amount of photons emitted per second. The unit is μmol/s
- From the amount of photons that hit one square metre in one second. The parameter here is PPFD (Photosynthetic Photon Flux Density). The unit is µmol/m2 · s
- The Photosynthetic Photon Efficacy (PPE). This indicates how efficient lighting is when it converts electrical energy into photons in the PAR range. The unit is µmol/J
For example, tomatoes require a photosynthetic photon flux density or PPFD of between 300 and 500µmol/m2 · s, and lettuce between 150 and 300µmol/m2 · s.
Per square metre, high-intensity photon presence usually results in more plant growth through photosynthesis, but this process is limited and depends on the amount of carbon dioxide the plants can absorb and process. A different logarithmically-shaped curve applies to each plant species.
When using LED lighting, there is a great deal of optical and electrical adjustments you can make to improve yields or influence growth phases. Creating the right light formula is a complex business.
To support users, light-emitting diode manufacturers provide online tools to compile light formulas. These tools can typically take information such as the input current and the number of LEDs used to calculate the full spectrum, photosynthetic photon flux (PPF), and wavelength colour ratios.
LED-based plant light systems have also been developed for R&D purposes. Researchers and farmers can use them in the lab or in climate chambers to develop new plant-specific light and growth formulas that later produce the ultimate properties such as quality, quantity and ingredients.
Brief Horticultural Glossary
- Red light (630 - 660 nm) is an important wavelength for photosynthesis and is primarily responsible for length growth. Red light also affects flowering, the resting phase, and germination
- Blue light (400 - 520 nm) is another important wavelength for photosynthesis, but must be mixed with other wavelengths because too much blue can inhibit growth. This wavelength is also associated with influencing chlorophyll concentration, increasing side bud growth and leaf thickness
- Dark red light (720 - 740 nm) from the IR spectrum influences germination and is said to shorten the flowering stage of plants and promote length growth
- UV light (280 - 400 nm) is in the research phase. With their cell-damaging properties, some plants such as lettuce and tomato are resistant to these wavelengths. Studies suggest that these wavelengths can be used to combat fungi. UV light may be responsible for the formation of antioxidants and polyphenols, which are important for plant nutrition
- Wavelength (λ, nm) – indicates the wavelength of the light emitted by the LED
- Photosynthetically Active Radiation (PAR, 400 nm – 700 nm) – the wavelength range of light that plants need for photosynthesis. This number can sometimes be misleading, as in this range all wavelengths are considered equally important for photosynthesis, although red and blue are the main drivers of photosynthesis. This means that the wavelengths from a green LED can be within the PAR range, but only have a limited impact on plant growth
- Photosynthetic photon flux (PPF, µmol-s-1) – quantifies the total amount of photosynthetically active photons produced per second by LEDs. Although it may seem strange for an electrical engineer to quantify the performance of a light source based on the number of photons emitted, keep in mind that photosynthesis is a biochemical process quantified by the number of sugar molecules produced per number of photons
- Photosynthetic photon flux density (PPFD, µmol-m-2-s-1) – quantifies the total amount of photosynthetically active photons reaching the monitored surface per second. This parameter is highly dependent on the distance and angle from the source. This is usually measured with a quantum meter that only responds to PAR wavelengths
- Photon efficacy (µmol·J) – this parameter indicates how efficient the LED is in generating PPF per joule of the electrical energy used
- Wall-plug efficiency (WPE, %) – this is defined as the energy conversion efficiency, a ratio of electrical power to optical power
- R-B ratio – the ratio of red to blue light emitted by the LED system